top of page
Project 1. Mechanisms that establish neuronal identities in the neocortex
The neocortex is the most differentiated structure in the central nervous system (CNS), which comprises of diverse glutamatergic neurons that are orderly positioned across laminar and areal compartments (Fig.1). Cytoarchitectonic changes between layers and areas are a general feature of the neocortex, and have been observed in mammals ranging from marsupials to primates. Intriguingly, the transitions from one cortical area to the next are often abrupt, and can be identified by sharp boundaries. This is reflected by dramatic shifts in both the thickness and numbers of neurons within layers at the transition point between functional areas. Therefore, to fully elucidate how architectonic differences between cortical layers and areas are generated, requires the understanding of the mechanism by which distinct layer neurons are specified and segregate within functional compartments at cellular resolutions. Developmentally, cortical glutamatergic neurons are generated from progenitors of the dorsal telencephalon, which undergo asymmetric cell divisions to produce different layer subtypes over a prolonged period of time. Furthermore, studies have shown that both mouse- and human-derived induced cortical progenitors recapitulate in vitro the sequential generation of principal layer subtypes that occurs in vivo: preplate, deep-layer (DL) and upper-layer (UL) neurons. These studies imply that a cortex-intrinsic program that regulates progenitor cell competence may drive the transitions between temporally discrete layer subtypes in the mammalian neocortex. However, the molecular mechanisms by which distinct cell fates in the cerebral cortex establish have only begun to unravel over the past years. Therefore, our efforts have been directed towards understanding how distinct neuronal subtypes are generated from a restricted pool of progenitor cells, focusing on the temporal regulation of cortical neurogenesis.
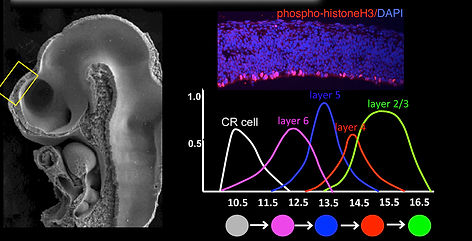

Project 2. Laminar- and area-specific control of neuronal differentiation and integration
Neocortical assembly is a highly dynamic process that requires multiple layers of regulation in cell behavior at the progenitor and postmitotic cell stages. The patterns of birth and migration of cortical projection neurons are considered to conform the following rules: each layer of neurons arises from the VZ and SVZ progenitors and moves radially toward the pial surface via multi-step guided migration processes; however, little is known about how the terminal positioning of neuronal subtypes is established. In this context, we found that Robo1, a member of the family of Roundabout receptors, regulates the radial dispersion of UL neurons in the neocortex. In a series of knockout and knockdown studies, the suppression of Robo1 was shown to result in E15-born neurons predominantly localizing to the uppermost part of layers 2/3, in contrast to control cells that were distributed radially in these layers. The sequential electroporation of fluorescent reporter constructs revealed that Robo1-suppressed cells fail to establish the characteristic inside-out neuronal distribution and accumulate beneath the marginal zone, also resulting in a thinner CP, as observed in Robo1 knockouts. Temporal analysis also reveals that E14.5-born cells, unlike E15.5 or E16.5 neurons, do not exhibit changes in their positioning upon Robo1 suppression. As the majority of E14-born neurons adopt a layer 4 fate and normally do not express detectable levels of Robo1 , these results imply that Robo signaling acts in a subtype-restricted manner, where layer 4 neurons are refractory to loss of Robo1 expression. The emerging picture of neocortical assembly is that while the identities of neuronal subtypes are largely determined at birth, the mechanisms by which these neurons are navigated to their final positions involve cell type- and context-dependent combinatorial codes that enable their precise integration into the neocortical circuit. We are currently using genetic fate-mapping and loss-of-function approaches to assess the patterns of neuronal migration and integration across layers and areas.
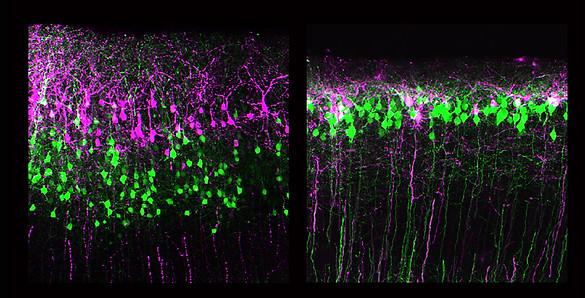
Loss of inside-out layer formation of neocortical layers II/III neurons in Robo1 suppressed neurons. In control shRNA transfected neurons, late-born pyramidal cells electroporated with DsRed at E16.5 (magenta) are able to migrate past earlier-born cells (green), resulting in an inside-out neuronal distribution. In Robo1 suppressed cells, neurons fail to undergo birthdate-dependent segregation of these neurons.
Project 3. Cell-cell communication in the establishment of neocortical territories
While the specification of neocortical subtypes and early patterning of the cortical primordium largely depends on cell intrinsic mechanisms, regional character of the neocortex has also been shown to be plastic, where positional cues provided by extrinsic sources are important for establishing and refining the boundary of neocortical areas. Indeed, cell-cell interactions such as intracortical excitatory and inhibitory connections, and thalamocortical innervation, accounts for the vast majority of synaptic inputs during the early phase of corticogenesis. However, the dynamics of these connections during development and the precise roles of early inputs on neocortical assembly remain open questions. Therefore, our aim is to examine the developmental process of specific intracortical and thalamocortical connections at cellular levels, and correlate them with the distribution and segregation patterns of layer neurons within distinct neocortical areal territories. Based on these connectome data, we are exploring their contribution to the establishment and refinement of cortical areas through loss-of-function approaches and manipulation of connectivity patterns. Together, these experiments will allow us to investigate the mechanistic basis by which the cytoarchitecture and functional circuitry develop in the human neocortex.

bottom of page